Fleas (Siphonaptera) - Factsheet for health professionals
Disclaimer: The information contained in this fact sheet is intended for the purpose of general information and should not substitute individual expert advice or the judgement of healthcare professionals.
Hazard associated with flea species
Current issues
Fleas are ectoparasitic blood-sucking insects with the ability to jump, which commonly infest wild and domestic animals (mainly dogs and cats) but also humans. In addition to serious dermatological conditions mainly related to allergic reactions, fleas can be vectors for various pathogens. There are more than 2 500 flea species in approximately 15 families [1]. The three most important flea species, in terms of global public health and veterinary relevance, are the house flea (Pulex irritans), the oriental rat flea (Xenopsylla cheopis), and the cat flea (Ctenocephalides felis). The chigoe flea or jigger (Tunga penetrans) is limited in distribution to Central and South America, Africa and the Indian sub-continent.
Biting and disease risk
None of the above-listed species have humans as their preferred host, but several animal-associated fleas, mainly from synanthropic (e.g. rodents) and domestic mammals (e.g. pigs, dogs, cats), may be found on humans and are capable of transmitting disease agents. Diseases which are relevant for Europe include plague (caused by Yersinia pestis), murine typhus (caused by Rickettsia typhi), flea-borne spotted fever (Rickettsia felis), and cat scratch disease (Bartonella henselae). Other animal diseases may be also transmitted by fleas: myxomatosis and Trypanosoma nabiasi in rabbits (by Spilopsyllus cuniculi), Bartonella quintana (by C. felis) or caprine mycoplasmal polyarthritis (via ‘goat fleas’) [2]. Additionally, fleas can serve as intermediate hosts for tapeworms. Dipylidium caninum (a typical cestodes of dogs, cats and, in some accidental cases, humans) is transmitted by ingesting fleas infected with the larval stages of Ctenocephalides spp. Similarly, Hymenolepis nana (of rodents) and Hymenolepis diminuta (of rodents and humans) develop in flea intermediate hosts (Nosopsyllus, Xenopsylla) [2].
Fleas are also considered to be a nuisance as they are able to cause allergic reactions and the site of the bite is pruritic.
Invasive species/global dispersion
Most fleas with public health and veterinary relevance have a global distribution, as they are associated with hosts that are also cosmopolitan. Their global dispersion is mediated by humans via transport and the trade of livestock, pets, and synanthropic rodents, such as mice and rats.
Ecological plasticity
All adult fleas are obligatory blood-feeders. In contrast, immature stages are free-living detritus feeders and their survival and development time depend on environmental factors. Once reaching the adult stage, fleas must find a suitable (or ideally optimal) host. The global dispersal and flexibility in host choice of medically important flea species are indicative of ecological plasticity. As they all feed on hosts living in close contact with humans (e.g. pets, other domestic animals, such as pigs, and synanthropic rats), they easily colonise all anthropic habitats, urban or rural, natural or artificial [3]. Being a pet owner, living or working in environments with poor sanitary conditions or having poor personal hygiene are risk factors for flea infestations.
Geographical distribution
Brief history of spread and European distribution
Most researchers agree that the house flea, P. irritans, originates in South America from where it was introduced to Africa, Europe and Asia via transoceanic contact as early as 3 000 BCE [3] [4]. The cat flea, C. felis, originates from Africa or the Middle East, probably with the African wildcat Felis lybica being used as a natural original host. From there, via ancient human migration and dog and cat domestication (around 15 000 BCE and 7 500 BCE respectively), the species started spreading. This spread became more intensive and global in the fifteenth to nineteenth centuries during the period of great maritime exploration and trade routes [5]. Similarly, the rat flea, X. cheopis, spread globally via rat-infested ships. Although its exact origin is not known, the theory is that it came from Egypt, where its species type locality is situated [6].
Recent data on the European distribution of medically important fleas are very scarce, at least for X. cheopis. In a study on parasites in wild brown rats from the UK, Webster and MacDonald (1995) observed that 14% of the fleas were X. cheopis [7]. A few other studies have shown that between 21−30% of Rattus rattus and Rattus norvegicus from France and Cyprus were carrying X. cheopis [8,9].
In the case of P. irritans, the distribution and host spectrum data in Europe are richer than for X. cheopis, pointing to a pan-European distribution with wild and domestic carnivores, as well as backyard pigs or domestic ruminants, as the principal hosts. Infestation rates are 11−30% in ruminants, 78% in pigs and 1−57% in domestic dogs [10-12].
Ctenocephalides felis is the most common dog and cat flea worldwide and several studies have shown it is widely distributed across the whole of Europe (summarised by [13]), with higher prevalence in rural areas.
Future expansion
There are few modelling studies available for predicting the expected changes in flea distribution [14]. The future expansion of fleas is hard to predict for various reasons. The current distribution of the rat flea, X. cheopis in Europe is unknown, hence it is impossible to predict how and whether it will spread. The other two vector species (P. irritans and C. felis) are known to be widely distributed. In the case of P. irritans, the increasing population of one of its main hosts in Europe, the red fox Vulpes vulpes, in urban areas [15] may lead to an increased risk of its fleas spreading to humans. In the case of C. felis, the emergence and wide usage of powerful insecticides for pets is likely to decrease its prevalence in pet dogs and cats and reduce the risk of human infestation. However, factors such as the increase in international travel of domestic dogs and cats, societal and lifestyle changes, wildlife urbanisation and climate change could potentially contribute to more flea infestations being found on humans [14].
Entomology
Species name/Classification: Siphonaptera: Pulicidae (Pulex irritans, Xenopsylla cheopis, Ctenocephalides felis)
Common names: House or human flea (Pulex irritans), Oriental or tropical rat flea (Xenopsylla cheopis), cat flea (Ctenocephalides felis)
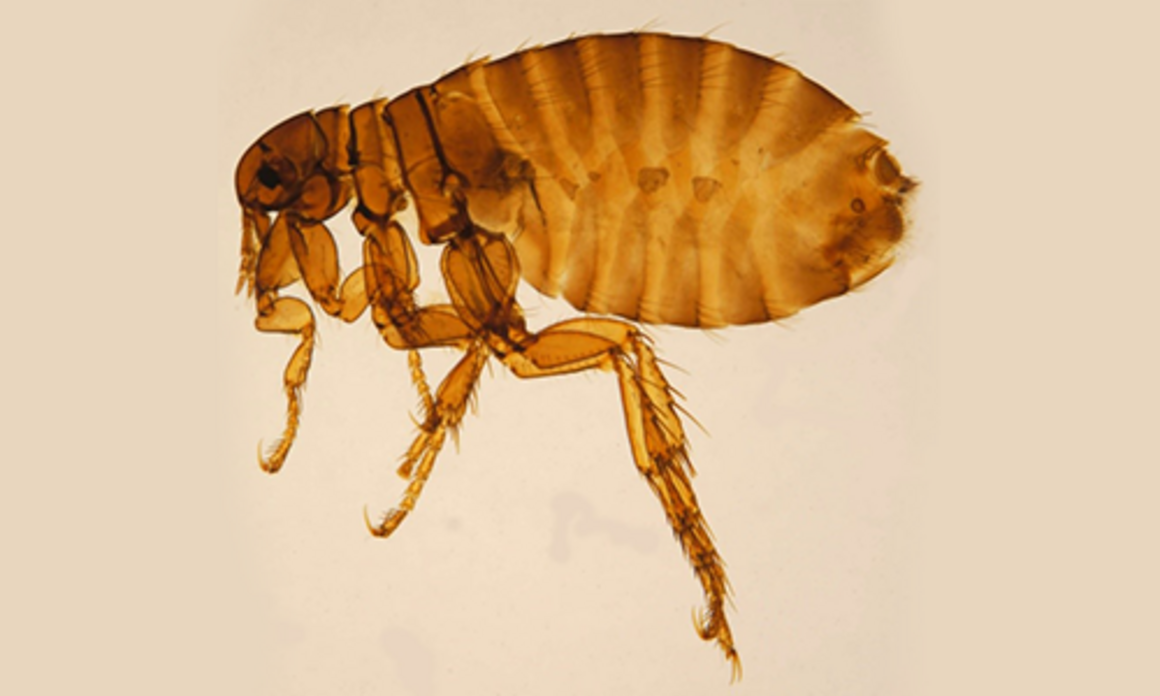
Morphological characters and similar species
All fleas belong to the order Siphonaptera, which includes around 2 500 species. Only two families include species of medical and veterinary importance: Pulicidae and Ceratophyllidae. The most important species found on domestic animals and/or humans are: C. felis (cats, dogs, on rare occasions also other domestic hosts and humans), Ctenocephalides canis (dogs, on rare occasions also other domestic hosts and humans), P. irritans (most domestic animals and humans) (Figure 1), X. cheopis (rats and humans) (Figure 2), S. cuniculi (rabbits), Echidnophaga gallinacea (chicken, dogs, humans), Ceratophyllus gallinae (chicken, on rare occasions also cats and human), T. penetrans (humans, pigs, dogs, cattle) [16].
Fleas are insects in which the adults are adapted to ectoparasitism, while their larval stages live in the environment. Larvae and adults are morphologically very different (Figures 1–3). Adult fleas have a laterally flattened body, adapted to movement by jumping, with long hind legs. They are usually small, the largest species only measuring up to 6 mm. The limits between the three body segments are hardly visible. The head is small and the abdomen is large [16]. Flea larvae look like tiny worms about 2–5 mm long, with a whitish body and pale-coloured hairs.
The main morphological characteristic used in flea classification is the presence of genal and/or pronotal combs; both genal and pronotal combs are present in C. felis, but absent in P. irritans and X. cheopis (see Figures 1–2). The latter two species are morphologically similar. This means that identification is challenging and requires experience. Specimens need to be mounted and only then can the species be distinguished by the location of the ocular bristle and the shape of the spermatheca.
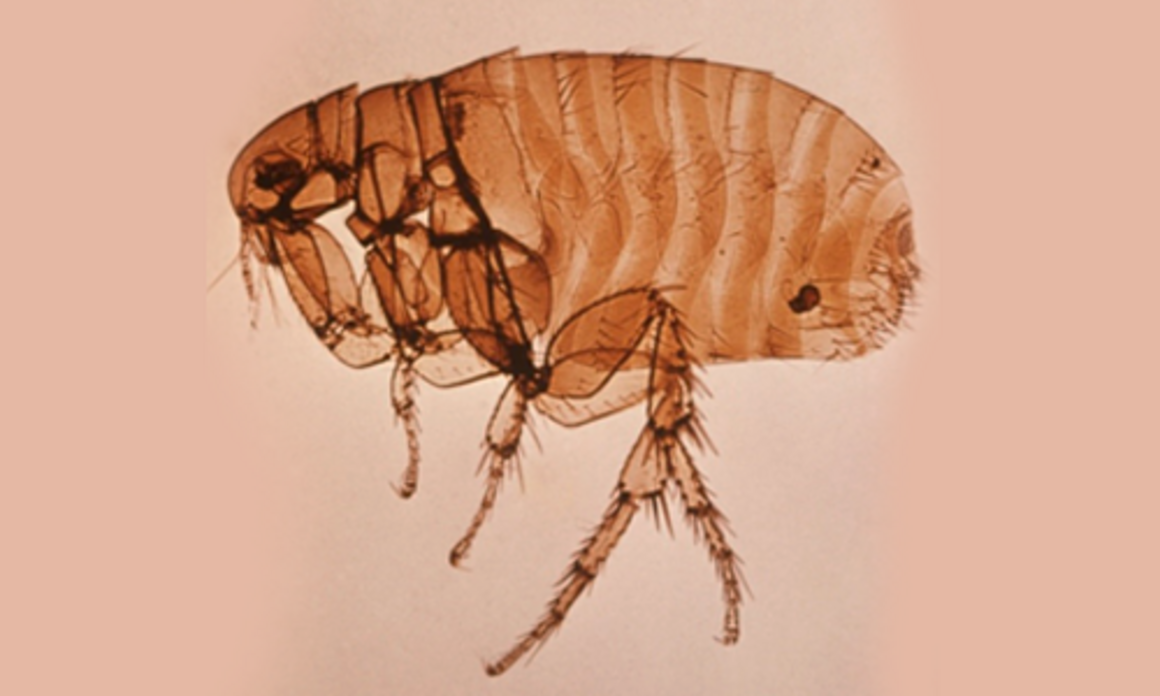
Development and life cycle
Fleas are holometabolic insects, which means that they undergo a complete metamorphosis including four life stages: egg, larva, pupa and imago or adult. Female fleas lay eggs scattered around the environment. The first instar larvae (L1) hatch after one week. The larvae feed on detritus, including faeces of adult fleas in some species, but also on flea eggs (cannibalism) and they moult twice (until L3). The development of larvae can take seven to 11 days under optimal environmental conditions, which are different for each flea species. The completely developed L3 produces a cocoon in which the pupa forms. The pupal stage lasts for 10 to 14 days and produces the adults. The development from egg to adult can take three to four weeks [16] or even longer.
Adult feeding behaviour
Both male and female adult fleas are hematophagous (i.e. blood feeders). In general, adult fleas do not actively seek the hosts. Instead, they prefer to remain motionless until the host approaches. They detect this approach by the sudden rise in temperature or humidity, or by detecting the vibrations caused by the host’s movement [17].
Adult fleas feed either directly from blood vessels or from blood pools produced by the bite of the mouthparts. Female fleas require a blood meal before maturing their eggs. During feeding, the skin of the host is cut using a specific structure of the mouthparts, by labral and lacinial stylets with several rows of teeth (mandibles are absent). The size of the blood meal taken during a single feeding event varies between species and even between males and females of the same species. For example, X. cheopis engorge ca. 0.1−0.2 µl blood at each feeding [3] while C. felis can ingest more than 13.6 µl [18]. The length of feeding also varies between species from as little as 2−5 minutes in Ctenocephalides felis to more than 10 minutes in X. cheopis. The frequency of feeding is also highly variable between species: Pulex irritans feeds every 2.5 hours while X. cheopis feeds twice per day [3].
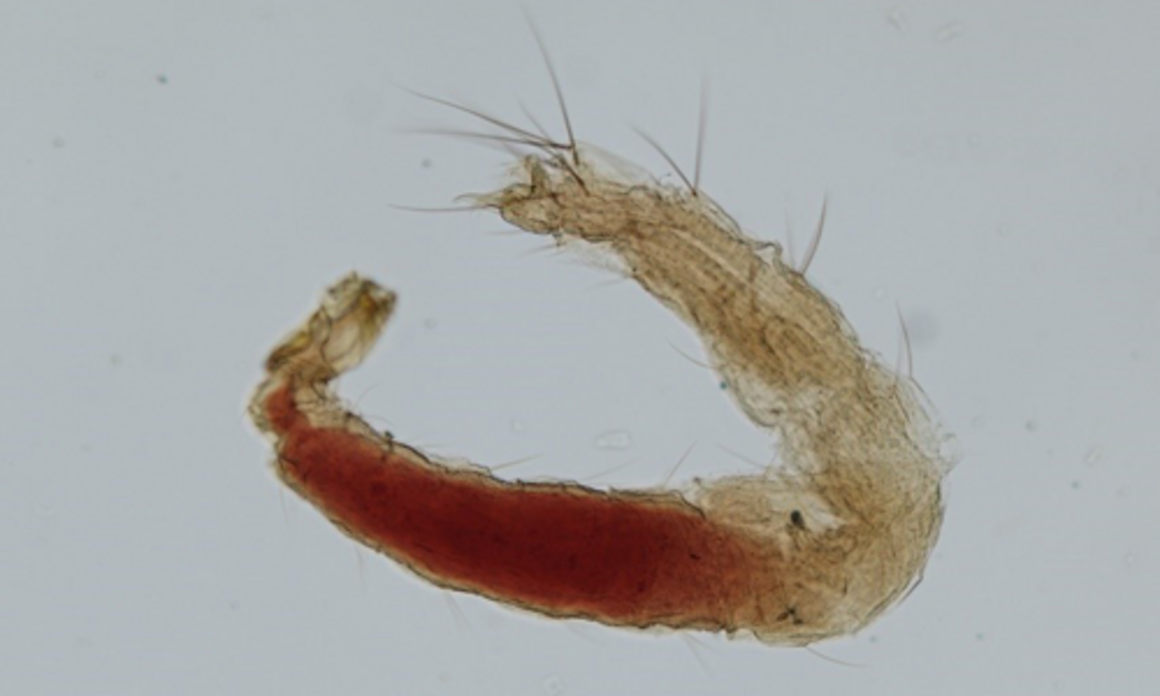
Reproduction and oviposition
After emerging from the pupa, both the males and the females generally require a blood meal for the activation of reproductive functions. Hence, mating can only take place after both sexes have fed (with a few exceptions, when mating occurs before the first blood meal). Mating occurs on- or off-host, depending on the species [3]. In C. felis, the females start laying eggs 24−48 hours after the first blood meal and they lay around 30 eggs per day, daily, for 50−100 days [16].
Activity
Flea prevalence (i.e. percentage of positive hosts from the total host population) and intensity (number of fleas per host) follow a seasonal pattern. The seasonality (variation of prevalence and intensity) varies with flea species. This is influenced mainly by flea survival and reproduction rates which depend on a combination of factors, such as climatic conditions (for the development and survival of the immature stages; survival of adults without a blood meal) and host density and availability [3].
In Europe, the seasonal variations in flea prevalence, intensity and reproductive patterns are significant due to the marked seasonal variations in temperature and precipitation [3]. However, due to their presence in anthropic environments, species such as X. cheopis or C. felis might show a less marked seasonal variation [3,13]. For P. irritans, higher prevalence and intensity have been reported on goats during the summer [11].
Host preference
Although flea species might prefer certain hosts, they will feed on any available host. For some flea species, survival and fertility is highly dependent on the host species [17]. The blood digestion rate greatly varies, even in the same flea species, depending on the host used for feeding and the amount ingested. For P. irritans, the off-host survival time varied from as little as two days after feeding on guinea pigs to two months after feeding on rabbits [3].
Pulex irritans has been found on a wide range of domestic and wildlife hosts, including humans. Despite its common name of house flea, P. irritans is more often found on pigs than on humans, the theory being that pigs were its original natural host in the Americas [17]. The cat flea (C. felis) is most commonly found on dogs and cats, but a large variety of other mammals, including humans, can serve as accidental hosts [13]. The rat flea (X. cheopis) feeds on many types of rodents, but occasionally other hosts, including humans, can be affected [19].
Epidemiology and transmission of pathogens
Vector status of different diseases of public health and veterinary importance
Plague
Plague is a severe zoonotic disease caused by Y. pestis, a Gram-negative rod-shaped bacterium. The pathogen has a wide-spread distribution, but the majority of human cases are reported from Africa, Asia and the Americas. The disease probably originates from Central Asia, with the first known human epidemic spreading from the Middle East to the Byzantine Empire, followed by the ‘Black Death’ pandemic during the fourteenth to the seventeenth century which caused significant human mortality in Europe. The last outbreaks in Europe or its immediate vicinity were reported in North-African Mediterranean ports (1943−1944), France and Italy in 1945. Outbreaks were reported in Algeria in 2003 [20] and in Libya in 2009 [21]. From 2010 to 2015 there were 3 248 cases reported worldwide, including 584 deaths [22]. In 2013, a large outbreak was reported in Madagascar, with more than 2 400 confirmed cases [23].
Yersinia pestis is maintained and spread among rodents by fleas. More than 200 rodent and approximately 20 lagomorph species have been reported to harbour the pathogen globally, with rats being the most important reservoirs in Europe. Although Y. pestis has been detected in more than 100 flea species, the main vector for humans are X. cheopis (worldwide), Xenopsylla brasiliensis (Africa, South America, India), and Xenopsylla astia (Southeast Asia) [1].
Outbreaks of plague are seasonal and associated with the ecology of rodents and their vectors. The most important risk factor is the exposure to fleas and rodent hosts in an area where the disease is active. Transmission of plague to humans can be via flea bites (infected fleas regurgitate the bacteria while feeding), contact with contaminated tissues (i.e. when handling infected animals) or through inhalation of infected aerosols (direct human-to-human transmission in the case of pneumonic plague). Infected cats and dogs can also transmit the disease to humans via bites or scratches. Humans develop the clinical disease after an incubation period of two to eight days [24].
Murine typhus
Murine typhus, also known as endemic typhus, is a bacterial zoonotic disease caused by Rickettsia typhi and is presently considered to be the most common flea-borne disease in humans. Murine typhus is found primarily in coastal regions, mainly in tropical and subtropical areas of the world, in association with high densities of rats which represent its wildlife reservoir [25]. Murine typhus is endemic in North Africa and the Middle East and also occurs in the Canary Islands, and on the island of Madeira. In continental Europe stable foci are present in Cyprus, Greece, Spain, Croatia and Bosnia-Herzegovina. In several other countries (France, Italy, North Macedonia, Portugal, Romania, Russia, Serbia, Slovakia, Slovenia) sporadic autochthonous cases have been reported [26].
People can become infected through an infected flea bite or contact (e.g. inhalation, conjunctival contact) with flea faeces. The main flea vectors of R. typhi are rat fleas (X. cheopis, Leptopsylla segnis) but also cat fleas (C. felis) and house fleas (P. irritans) can be involved in urban or sub-urban cycles, with possums and cats as pathogen reservoirs [1,25]. It has also been suggested that the fleas (Leptopsylla segnis) of other types of rat are potential vectors of R. typhi, but experimental proof is still needed [27].
Flea-borne spotted fever
Flea-borne spotted fever is a more recently described rickettsial zoonotic disease caused by R. felis which is responsible for an illness that is similar to, but less severe than murine typhus. Since its discovery in 1990 in a laboratory colony of cat fleas (C. felis) it has been recognised as an important cause of human disease worldwide [25]. In Europe, autochthonous cases have been detected in France (2009), Germany (1997), Spain (2006), and Sweden (2010) [26].
The main vector of R. felis is the cat flea, C. felis, but other flea species (X. cheopis, Archeopsylla erinacei) have be involved in the transmission or have been found to carry the pathogen [26,28]. Cat fleas are able to vertically transmit the pathogen for several generations. So far, no mammal reservoir is known for R. felis [25]. The DNA of R. felis has also been detected in other arthropods, such as ticks, mites, lice, and mosquitoes [29,30] but their role as reservoirs or vectors is unknown.
Cat scratch disease
Cat scratch disease is a bacterial zoonosis caused by B. henselae, the most common species of Bartonella found in humans. The main vector is C. felis, which is responsible mainly for maintaining the infection in cat populations. However, the most important infectious material is flea faeces, from where the bacteria infect wounds, such as cuts or scratches [13]. The name of the disease refers to the most common method of transmission to humans, by cat scratch when the claws of cats are contaminated with infected flea faeces. Serological studies in cats have shown higher antibody prevalence in warm, humid areas than in cold and dry areas, in feral cats compared to owned cats and in cats infected with fleas compared to flea-free cats [31].
Tularaemia
Tularaemia is a zoonosis caused by Francisella tularensis, a gammaproteobacterium. The disease is endemic in several areas of the northern hemisphere (Europe, Northern and Central Asia and North America). The clinical presentation in humans is complex, with several classical forms described.
Various vertebrates act as reservoirs of infection, with lagomorphs being the most often incriminated. The most common route of human infection is water-borne, but it can also occur via a tick or fly bite or direct contact during the handling of infected animal tissues. Other routes of infection are inhalation or ingestion. Other arthropods (fleas, lice, deer flies, mosquitoes, bedbugs, mites) have also been suggested as vectors, but their true role still needs to be clarified. Among fleas, several species have been suggested as possible reservoirs and/or vectors: X. cheopis, Ceratophyllus acutus, Ceratophyllus fasciatus [32].
Likelihood of introduction and establishment of flea-borne pathogen transmission cycles in Europe
There is currently no plague in Europe and the last reported cases occurred after the Second World War. The three most plague-endemic countries in the world are currently the Democratic Republic of the Congo, Madagascar, and Peru [22], so travellers and cargo transport from these countries should be considered as a potential risk of pathogen introduction to Europe. However, the highest importation risk to Europe may be posed by infected rats and rat fleas via trans-Mediterranean maritime routes since X. cheopis is prevalent in countries such as Algeria [33].
The likelihood of onward transmission from a traveller infected with bubonic plague via fleas is very low, as it requires the presence of competent flea vectors on the infected traveller or at the traveller’s destination. Both are unlikely as rat fleas do not live on people —they leave humans after feeding— and X. cheopis does not infest houses unless these are co-inhabited by rats [33]. There is no recent documentation in Europe of local transmission from imported cases of the bubonic form of plague. The main risk of local transmission is posed by travellers infected with pneumonic plague, who could directly spread the disease via respiratory droplets. It should be noted that untreated bubonic and septicaemic forms of plague may develop into pneumonic plague.
Due to the non-specific clinical picture, murine typhus is usually indistinguishable from other febrile conditions, mainly in tropical areas where it is underdiagnosed. Travellers entering or returning from endemic areas may also be misdiagnosed, except for the severe cases when specific tests are usually done [25]. Imported cases have been reported in many European countries [26], but with no onward local transmission events documented after importation. After being established in a human population, R. typhi can be also transmitted in a human-flea-human cycle by P. irritans and, experimentally, even by body lice (Pediculus humanus humanus) and become epidemic. In Europe, the highest risk is probably posed by the cat flea, C. felis which is present inside human habitats on a year-round basis [26]. Seroprevalence studies in people commonly exposed to rats and their fleas has shown the presence of low antibody levels [34].
Cat scratch disease has a worldwide distribution, including Europe. Although the disease is commonly subclinical and self-limiting, complications such as angiomatosis, endocarditis or systemic infection have been reported in vulnerable people, such as children and immunocompromised patients [31].
Tularaemia is endemic in Europe, but the sources of human infection vary in each country. Although infection can stem from a tick bite or direct contact with aerosols, it is also commonly considered to be a water-borne infection [35]. In addition, in a number of countries it has been associated with direct contact with infected hares during hunting sessions (Czechia, France, Germany, Slovakia, Spain), contamination of food or water by infected rodents (Bulgaria, Kosovo, Norway, Serbia, Turkey) or mosquitoes (Sweden) [32,35]. The role of fleas in the transmission to humans in Europe seems to be limited compared to that of ticks and biting flies.
Public health control measures
The presence of fleas in human environments is more or less ubiquitous, although not easily recognised. Cat fleas are common on pet and stray cats and dogs and can be a nuisance for people in frequent contact with pets. General control measures for fleas may include periodical application of ectoparasiticides on pets, cleaning and disinfection of the pet’s environment (e.g. pet beds, floor) to eliminate larvae; control of stray dog and cat populations and rodents in populated areas and public awareness to educate people about avoiding contact with rodents. More specific measures for flea-borne diseases are listed below.
Surveillance and prompt diagnosis of plague cases in humans is essential to prevent onward transmission [1]. One of the suggested control measures for preventing the introduction of plague into Europe via rodents and their fleas is the implementation of rodent-control programmes at points of entry, such as harbours [36] and in urban areas [1], combined with vector control activities.
Following the high incidence of murine typhus after the Second World War, drastic rat and vector control programmes caused a significant drop in human cases [25]. Therefore similar measures to those described above could be implemented for plague in risk areas.
As the main vector and reservoir of R. felis is C. felis, the main control measures can focus on the control of cat fleas, as mentioned above. As no mammalian reservoir hosts are currently known for R. felis, it is not possible to control these fleas by targeting reservoir hosts.
Controlling flea infestation in cats is the only recommended measure that can reduce the likelihood of human infection with B. henselae [13].
Key areas of uncertainty
Flea-borne diseases are relatively neglected in Europe compared to other vector-borne diseases in terms of targeted surveillance, research and control. One of the main knowledge gaps in understanding the risks of pathogen transmission from fleas to humans is the lack of geographical distribution da,ta mainly for the house flea, P. irritans and the rat flea, X. cheopis. Moreover, there is little data available on the prevalence of most flea-borne pathogens in fleas, vertebrate reservoirs, and humans. Targeted and detailed studies are needed to fill these gaps.
Novel genotypes of flea-borne pathogens, particularly Rickettsiae, are continually being found in various insect vectors, including fleas, worldwide [25]. However, their specific taxonomic status, their eco-epidemiology and public health and veterinary significance requires more in-depth research. There should also be further study of ectoparasites and pathogens of rodents at points of entry in order to better understand the risk to public health.
References
- Russell RC, Otranto D, Wall RL. The Encyclopedia of Medical and Veterinary Entomology. Wallingford: CABI; 2013.
- Durden LA, Hinkle NC. Chapter 10 Fleas (Siphonaptera). In: Mullen GR, Durden LA, editors. Medical and Veterinary Entomology. 3rd edition. Cambridge (MA): Academic Press; 2019. p. 145-69.
- Krasnov BR. Functional and evolutionary ecology of fleas: a model for ecological parasitology. Cambridge: Cambridge University Press; 2008.
- Buckland PC, Sadler JP. A biogeography of the human flea, Pulex-irritans L (Siphonaptera, Pulicidae). J Biogeogr. 1989 Mar;16(2):115-20.
- Lawrence AL, Webb CE, Clark NJ, Halajian A, Mihalca AD, Miret J, et al. Out-of-Africa, human-mediated dispersal of the common cat flea, Ctenocephalides felis: the hitchhiker's guide to world domination. Int J Parasit. 2019 Apr;49(5):321-36.
- Harimalala M, Telfer S, Delatte H, Watts PC, Miarinjara A, Ramihangihajason TR, et al. Genetic structure and gene flow of the flea Xenopsylla cheopis in Madagascar and Mayotte. Parasites & Vectors. 2017 Jul;10:13.
- Webster JP, Macdonald DW. Parasites of wild brown rats (Rattus norvegicus) on UK farms. Parasitology. 1995 Sep;111 (Pt 3):247-55.
- Antoniou M, Psaroulaki A, Toumazos P, Mazeris A, Ioannou I, Papaprodromou M, et al. Rats as indicators of the presence and dispersal of pathogens in Cyprus: ectoparasites, parasitic helminths, enteric bacteria, and encephalomyocarditis virus. Vector-Borne Zoonotic Dis. 2010 Nov;10(9):867-73.
- Davoust B, Boni M, Branquet D, Ducos de Lahitte J, Martet G. [Research on three parasitic infestations in rats captured in Marseille: evaluation of the zoonotic risk]. Bulletin de l'Academie nationale de medecine. 1997 May;181(5):887-95; discussion 96-7.
- Beugnet F, Labuschagne M, Fourie J, Guillot J, Farkas R, Cozma V, et al. Occurrence of Dipylidium caninum in fleas from client-owned cats and dogs in Europe using a new PCR detection assay. Vet Parasitol. 2014 Sep;205(1-2):300-6.
- Christodoulopoulos G, Theodoropoulos G, Kominakis A, Theis JH. Biological, seasonal and environmental factors associated with Pulex irritans infestation of dairy goats in Greece. Vet Parasitol. 2006 Apr;137(1-2):137-43.
- Foley P, Foley J, Sandor AD, Ionica AM, Matei IA, D'Amico G, et al. Diversity of flea (Siphonaptera) parasites on red foxes (Vulpes vulpes) in Romania. Journal of Medical Entomology. 2017 Sep;54(5):1243-50.
- Rust MK. The biology and ecology of cat fleas and advancements in their pest management: a review. Insects. 2017 Dec;8(4):51.
- Beugnet F, Chalvet-Monfray K, Loukos H. FleaTickRisk: a meteorological model developed to monitor and predict the activity and density of three tick species and the cat flea in Europe. Geospatial Health. 2009 Nov;4(1):97-113.
- Plumer L, Davison J, Saarma U. Rapid urbanization of red foxes in Estonia: distribution, behaviour, attacks on domestic animals, and health-risks related to zoonotic diseases. PLoS One. 2014 Dec;9(12):15.
- Deplazes P, Eckert J, Mathis A, von Samson-Himmelstjerna G, Zahner H. Parasitology in veterinary medicine. Wageningen Academic Publishers; 2016.
- Wall R, Shearer D. Veterinary entomology: arthropod ectoparasites of veterinary importance: Springer Science & Business Media; 1997.
- Rust MK, Dryden MW. The biology, ecology, and management of the cat flea. Annu Rev Entomol. 1997;42:451-73.
- Mears S, Clark F, Greenwood M, Larsen KS. Host location, survival and fecundity of the Oriental rat flea Xenopsylla cheopis (Siphonaptera: Pulicidae) in relation to black rat Rattus rattus (Rodentia: Muridae) host age and sex. Bull Entomol Res. 2002 Oct;92(5):375-84.
- Bertherat E, Bekhoucha S, Chougrani S, Razik F, Duchemin JB, Houti L, et al. Plague reappearance in Algeria after 50 years, 2003. Emerg Infect Dis. 2007 Oct;13(10):1459-62.
- Tarantola A, Mollet T, Gueguen J, Barboza P, Bertherat E. Plague outbreak in the Libyan Arab Jamahiriya. Eurosurveillance. 2009 Jul;14(26):3.
- World Health Organization. Fact sheet: Plague: Available at: https://www.who.int/news-room/fact-sheets/detail/plague 2020 [cited 2021].
- Mead PS. Plague in Madagascar - A tragic opportunity for improving public health. N Engl J Med. 2018 Jan;378(2):106-8.
- Dillard RL, Juergens AL. Plague. StatPearls. Treasure Island (FL): StatPearls Publishing; 2020.
- Blanton LS, Walker DH. Flea-borne rickettsioses and Rickettsiae. Am J Trop Med Hyg. 2017 Jan;96(1):53-6.
- Portillo A, Santibanez S, Garcia-Alvarez L, Palomar AM, Oteo JA. Rickettsioses in Europe. Microbes Infect. 2015 Nov-Dec;17(11-12):834-8
- Azad AF, Traub R. Transmission of murine Typhus rickettsiae by Leptopsylla segnis (Siphonaptera, Leptopsyllidae). Journal of Medical Entomology. 1987 Nov;24(6):689-93.
- Gilles J, Just FT, Silaghi C, Pradel I, Passos LMF, Lengauer H, et al. Rickettsia felis in fleas, Germany. Emerg Infect Dis. 2008 Aug;14(8):1294-6.
- Brown LD, Macaluso KR. Rickettsia felis, an emerging flea-borne rickettsiosis. Curr Trop Med Rep. 2016;3:27-39.
- Socolovschi C, Pages F, Ndiath MO, Ratmanov P, Raoult D. Rickettsia species in African Anopheles mosquitoes. PLoS One. 2012 Oct;7(10):8.
- Guptill L. Feline bartonellosis. The Veterinary clinics of North America. Small animal practice. 2010 Nov;40(6):1073-90.
- Telford SR, Goethert HK. Ecology of Francisella tularensis. In: Douglas AE, editor. Annu Rev Entomol. Annual Review of Entomology. 65. Palo Alto: Annual Reviews; 2020. p. 351-72.
- Beaucournu J-C, Launay, H. Les puces de France et du bassin méditerranéen occidental. Faunes de France 76. Fédération francaise des sociétés de sciences naturelles (ed.) 1990. p. 548.
- Badiaga S, Benkouiten S, Hajji H, Raoult D, Brouqui P. Murine typhus in the homeless. Comp Immunol Microbiol Infect Dis. 2012 Jan;35(1):39-43.
- Hennebique A, Boisset S, Maurin M. Tularemia as a waterborne disease: a review. Emerging Microbes & Infections. 2019;8(1):1027-42.
- VBORNET. WP 4.2 – Risk assessment of diseases transmitte